Induced pluripotent stem cells (iPSCs) have become a mainstay in disease modeling and drug development, offering almost unlimited opportunities to study human biology in the lab. However, working with these cells can present hurdles that catch even the most seasoned cell biologist off guard. Whether you’re new to iPSC culture or looking to learn more about them, understanding their challenges will help boost your success in the lab! In this first instalment of our news iPSC series, we’ll present some of the major issues you’re likely to face when working with iPSCs and share practical tips to address them.
The impact of cell variability
Cell variability or heterogeneity means that cells within a population differ in certain properties, for example, shape, size, position in the cell cycle, or molecular-level characteristics such as expression of cell-surface receptors. While variability occurs in all cell types and has its benefits in nature, it poses challenges in the laboratory, e.g., with maintaining consistent experimental conditions, ensuring quality control, and producing reliable results. When working with iPSCs and iPSC-derivative cell types that are differentiated and lineage committed, it is crucial to minimize variation because it can affect differentiation potency, lineage commitment, morphology, gene and protein expression patterns, all of which can impact the cells’ ability to recapitulate the phenotypic properties of the disease being studied, or the reliability of drug screening assays.
Where does the variability come from?
Within iPSCs, cell variability comes from three main sources:
- Interdonor variability. This stems from differences between donor samples, i.e., the inherent genetic and chromosomal differences that exist between humans. In some cases, iPSCs derived from blood samples behave differently from iPSCs derived from skin samples from the same donor; epigenetics is one possible explanation for this observation.
- Interclonal variability. Variability can arise in clones that are generated from the same donor, but this can be addressed by pooling the clones during the iPSC reprogramming phase to select for the most optimal culture for biobanking.
- Intraclonal variability. Differences may exist between batches of the same clone, but this should not have a major impact on your experiment. In our experience, iPSCs from the same donor sample and subjected to the same reprogramming procedure should have little-to-no-variation if cell culture conditions are well maintained.
Within iPSC-derived cell types, variability usually comes from the following sources:
- Cell populations can contain a mixture of developmental stages, including progenitors, differentiated cells, and mature cells, and the proportions of these subpopulations may vary between experiments. This variability can potentially affect results and their interpretation. Importantly, confusion often occurs between cellular maturity (which can be achieved in vitro) and complete functional maturity (which requires the physiological, genetic, and environmental conditions within the body).
- Donor-to-donor variation. This applies to patient-derived cell lines of any disease. As an example, iPSC-derived motor neurons from ALS patients can show variabilities in functional and morphological properties, e.g., in their neurite formation patterns. Such differences make sense given the natural variation across ALS patients, including different disease profiles, age and gender differences, genetics/mutations and age at onset.
- Gene-editing of iPSC-derived clones often leads to differentiation variabilities.
Reproducibility challenges:
iPSCs and iPSC-derivative cell types are known for their high sensitivity to in vitro cell culture conditions and require specialized media. Some of the key considerations for iPSC culture media and practice include:
Media composition. Defined, serum-free formulations are essential to maintain pluripotency and ensure reproducible results. Fetal bovine serum (FBS), which is rich in hormones and growth factors, is commonly used as a media supplement to boost cell proliferation and growth. While some researchers have reported that FBS can augment the efficiency of iPSC generation from human adipose-derived stem cells (1), the drawback of FBS is its undefined nature. In our experience, the addition of FBS to culture media is a major source of variability in efforts to biobank and expand iPSCs, and furthermore, it is not permitted under clinical Good Manufacturing Practice (cGMP) processes.
Environmental conditions. iPSCs are sensitive to minute fluctuations in temperature, humidity, atmospheric oxygen, and CO2 levels. These conditions and atmospheric conditions in the lab in general must be observed/tracked and controlled (if possible) to minimize variation and improve reproducibility.
Timing of media changes. Given the sensitivity of iPSCs to environmental fluctuations, regular and consistent feeding schedules are necessary to maintain optimal conditions and minimize variability.
Handling techniques. Standardized passaging methods do not work well for iPSCs. Procedures involving Trypsin/EDTA should be abandoned and “over-passaging” is highly discouraged despite notions that it can be cost-effective; it won’t be useful if the iPSCs or iPSC-derivative cell type trans-differentiates or dies in culture.
Genetic instability. It is important to be aware that iPSCs are prone to genetic instability – they can acquire various chromosomal and genetic abnormalities during both the reprogramming process and subsequent cell culture. These changes are another source of variability that can affect cell behavior and experimental outcomes. Some considerations in this context when working with iPSCs include:
- The chromosomal abnormalities that can occur include single point mutations, copy number variations, translocations and aneuploidy (extra chromosomes). However, it’s important to remember that while some degree of genetic instability is inherent, the likelihood of major abnormalities and the extent to which they arise is influenced by the reprogramming method used. For example, if CRISPR is used to gene-edit the iPSCs, chromosomal instability may increase over time during subsequent reprogramming. Of note, the proto-oncogene c-Myc – which was one of the original genes used in reprogramming and whose use has reduced in recent years – strongly promotes iPSC generation, but it has also been shown to increase tumor formation in iPSC-derived chimeric mice (2).
- It’s well established that passage number affects genome instability and lineage commitment, and for reasons that are still unclear, “over-passaging” can impact cell identity. As a rule of thumb, iPSC clones should not be passaged more than 20 times. Another source of genetic instability is that the donated sample may have inherent chromosomal abnormalities. This possibility can be addressed by comparing experimental results from several donor samples, which should account for the natural genetic variation between individuals.
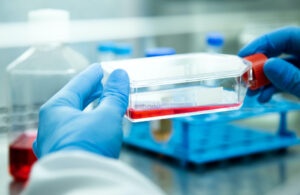
Culturing human iPSCs and iPSC-derivative cell types.
iPSC differentiation is not always straightforward!
iPSC differentiation is a very complex topic that would be impossible to address in detail in a single article. Some of the most notable challenges include:
- The low efficiency of somatic cell conversion to iPSCs can make iPSC reprogramming very resource-intensive and time consuming. This varies with cell type and with method of differentiation.
- It is necessary to develop differentiation protocols for each cell type which exacerbates the costs of time and resources used.
- Even when you have used specialized media, controlled environmental conditions and handled the cells extremely carefully, iPSCs don’t always mature as expected! Sometimes, you need the 3D organoids to be transplanted back to the human/animal for full functional maturity. For example, human kidney organoids may develop basic structures in vitro, but they need the complex physiological environment of a living body to carry out mature kidney functions such as blood filtration and hormone regulation.
- Researchers have shown that iPSCs can ‘remember’ epigenetic marks from their cell of origin and this may influence their differentiation potential and behavior (3). While you should be aware that this property might pose challenges when studying certain diseases or when generating certain desired cell types, the impact of genetic differences between donors and patients is much more significant.
Spontaneous differentiation can occur
- Yes, that’s right! iPSCs can spontaneously differentiate in culture, affecting culture purity and reproducibility. While the underlying mechanisms are not fully understood, this happens because cultured iPSCs exist in a fine balance between maintaining pluripotency and being ready to differentiate, which is governed by complex networks of gene expression and regulation. Even minor changes in culture conditions can disrupt those networks and trigger differentiation programs.
- You can mitigate the risk of spontaneous differentiation by regularly (i.e. daily) monitoring and feeding the cells. Make sure to check the pH of your cell culture media and be careful not to over-passage the cells.
- Spontaneous differentiation can also occur when cell “density” is wrong – for iPSCs and for iPSC-derivative cell types, or just during reprogramming and differentiation. Density matters. For example, you might have too many iPSC-derived neurons in a dish so that they become overcrowded leading to suboptimal culture conditions. On this note, some iPSC-derived glial cell types have high intrinsic metabolism and overcrowding is very bad for them in culture. When overcrowded, these cells actually try to float and escape!
Stay tuned for more articles about iPSCs!
While we have tried to address the major challenges and provided tips to address them in this article, there is no one-size-fits-all guide to suit all iPSC-derived cell types.
In practice, scientists working with iPSC-derived cells should be aware that certain cell types in their differentiated state (e.g., neurons or liver cell types) have limited growth and expansion capacity. Similarly, it’s important to have reasonable expectations as to what kinds of functional or target-driven assays are appropriate for any iPSC-derived cell type of interest – and you can stay tuned to learn much more about this in our next article!
References:
- Kwon D, Kim JS, Cha BH, et al. The Effect of Fetal Bovine Serum (FBS) on Efficacy of Cellular Reprogramming for Induced Pluripotent Stem Cell (iPSC) Generation. Cell Transplant. 2016;25(6):1025-42.
- Nakagawa M, Takizawa N, Narita M, et al. Promotion of direct reprogramming by transformation-deficient Myc. Proc Natl Acad Sci U S A. 2010 Aug 10;107(32):14152-7.
- Kim K, Doi A, Wen B, et al. Epigenetic memory in induced pluripotent stem cells. Nature. 2010 Sep 16;467(7313):285-90.
Karen O’Hanlon Cohrt is an independent Science Writer with a PhD in biotechnology from Maynooth University, Ireland (2011). After her PhD, Karen relocated to Denmark where she held postdoctoral positions in mycology and later in human cell cycle regulation, before moving to the world of drug discovery. Karen has been a full-time science writer since 2017, and has since then held numerous contract roles in science communication and editing spanning diverse topics including diagnostics, molecular biology, and gene therapy. Her broad research background provides the technical know-how to support scientists in diverse areas, and this in combination with her passion for learning helps her to keep abreast of exciting research developments as they unfold. Karen is currently based in Ireland, and you can follow her on Linkedin here.