Welcome back to our blog series about the blood brain barrier (BBB). In Part 1, we provided a brief history on the discovery of the BBB, and introduced what we currently know about its composition and biological functions. In Part 2, we explain why reliable BBB models are critical for drug development and summarize the currently available in vitro BBB models.
As described in Part 1, the BBB effectively separates the brain from the rest of the body, and in doing so, it prevents the passage of pathogens, drugs, toxins, and other potentially harmful substances from the bloodstream into the brain.
While the essential barrier function is pivotal to protecting the brain, it also poses a major challenge to the therapeutic intervention of diseases of the central nervous system (CNS), by blocking the entry of drugs into the brain. Given that most of what we know about the human BBB is extrapolated from studies in non-rodent or even non-mammalian animal models and cell lines, it is hardly surprising that the high failure rates of experimental CNS drugs in clinical trials is in part due to our limited understanding of the BBB, including drug permeability at the BBB, how drugs distribute once within the brain, and therapeutic target engagement in the brain.
Reliable human BBB models are critical for CNS drug development
As the pharmaceutical industry advances with novel therapeutic modalities that have the potential to treat or even cure certain brain diseases, e.g., adeno-associated virus (AAV)-mediated gene therapies, CAR-T cell therapies, and therapies based on CRISPR and other gene-editing technologies, the demand for a reliable human BBB model has never been greater. A more complete understanding of the molecular composition of the human BBB should improve the outcomes of CNS drug development and contribute to the development of safe and effective brain therapies by:
- Allowing drug developers to test the permeability of potential drug candidates across the BBB in a reproducible way that translates to the in vivo human-relevant setting.
- Making it possible to reliably monitor the therapeutic efficacy and toxicity of novel CNS drug candidates prior to clinical trials.
- Uncovering the mechanisms underlying diseases caused by damage to the BBB, e.g., stroke, multiple sclerosis (MS), amyotrophic lateral sclerosis (ALS), Parkinson’s disease (PD), and other neurodegenerative disorders. This will help to identify new therapeutic targets for these diseases.
The current landscape of in vitro BBB models
Today, a number of in vitro models designed to mimic the BBB are used to study barrier function, both to screen new CNS drug candidates for BBB permeability and to shed light on how the BBB is regulated. Of these, the transwell assay is the most widely used.
The Transwell Assay
The classical transwell assay is based on a static apparatus in which a confluent monolayer of brain microvascular endothelial cells (BMECs) is cultured on a porous membrane that separates two chambers; an apical (on top) chamber and a basolateral (on the bottom to the side) chamber (see Figure 1).
In earlier years, transwell apparatuses were primarily used for monoculturing either endothelial or epithelial cells, such as Caco-2 or MDCK cell lines, respectively. Today, co-culture is widely used since BBB function is highly dependent upon interactions between BMECs and supporting cells, including astrocytes, pericytes, and/or neurons, which together with the BMECs constitute the neurovascular unit (NVU).
BMECs are cultured on top of the membrane, while supporting cells are seeded in contact (on the other side of the membrane) or non-contact (in the basolateral chamber) positions below the membrane. The addition of culture media that has been conditioned by supporting cell types to the basolateral chamber may also be used to influence endothelial cell differentiation or upregulate barrier function. By measuring the transport of solutes or cells from the apical to the basolateral chambers, the transwell assay can provide clues about BBB permeability and the mechanisms behind drug/solute transport.
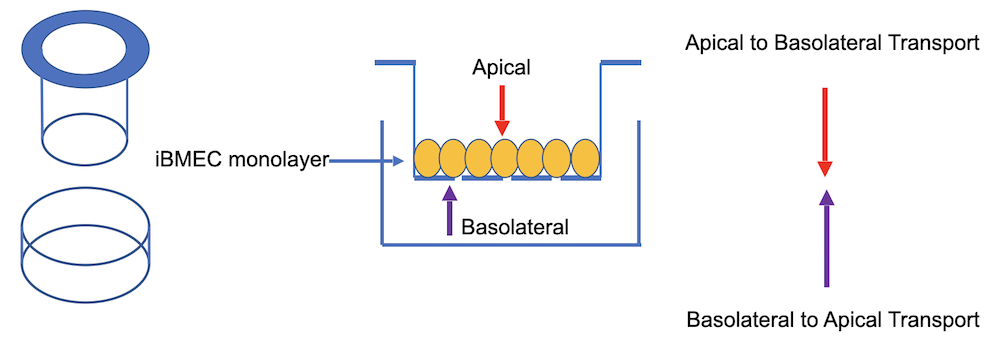
Figure 1: Schematic representation of the classical transwell assay. The apical and basolateral chambers, on either side of a monolayer of human iPSC derived brain microvascular endothelial cells (iBMECs) that are cultured on a porous membrane, are shown.
Transendothelial electrical resistance (TEER) and permeability assessments
The two most commonly used parameters for quantitative assessment of barrier function using the transwell assay are transendothelial electrical resistance (TEER) and permeability.
TEER studies provide a real-time measurement of the electrical resistance through the transwell membrane, which can provide an indication about membrane, ergo, BBB integrity. TEER is measured between two electrodes, where one is placed in the apical and the other in the basolateral chamber. TEER assessments are popular as they are easy to perform, and can be performed repeatedly at very short (e.g. second) intervals over several days to weeks without damaging cells, but they are not without limitations. The biggest drawback is arguably the dramatic variation in TEER values that occurs depending on which cell type is used, and even when the same cell type is used but from different sources (e.g., TEER values for primary BMECs are highly variable and drop in a passage-dependent manner). These variations pose challenges to reproducibility and greatly impede interlaboratory comparisons, as well as efforts to set industry standards.
Permeability assessments measure the ability of certain reporter substances to cross the membrane, e.g., high molecular weight mannose, sucrose, or dextrans, which are bound to a fluorescent dye. A substance is added to the apical chamber and its time-dependent concentration is measured in the basolateral chamber. The goals of TEER and permeability testing overlap, since they both measure passage of substances across a cell monolayer, however, a permeability study is typically performed only at a single time point during an experiment.
Benefits of the transwell assay include its cost-effectiveness, ease of use, high-throughput compatibility, and that the cells remain visible throughout the assay procedure. Its main drawbacks are its static and two-dimensional nature and that the cells cultured on the membrane are not exposed to blood flow. Furthermore, the environment within the transwell is devoid of shear stress, which is a physiological force generated by blood flow across the apical surface of vascular endothelium in the NVU, and which is needed for tight junction formation in BMECs. These shortcomings greatly limit the extent to which the transwell model recapitulates human BBB physiology.
Alternative in vitro platforms to study BBB function
While the transwell assay remains the most widely used in vitro BBB model, other platforms that solve some of its limitations exist and some of these are briefly mentioned here.
- Membrane-based and matrix-containing microfluidic models. These are designed to be a more dynamic version of the classic transwell assay and are generally considered to better represent the microenvironment of the BBB, although not all NVU cell phenotypes are recapitulated. The setup is somewhat similar to the classic transwell, but with a thicker porous membrane mounted into a microfluidic device, with or without the addition of an extracellular matrix (ECM) into the basolateral chamber. Culture media is added to the device via a pump to mimic blood flow and shear stress. When present, the ECM allows co-culture of supporting cell types in a 3D setting. PEER and permeability measurements can be undertaken.
- BBB-on-a-Chip. Chip-based versions of the microfluidic BBB models have emerged in recent years. These generate dynamic flow and gas exchange through pumping and gas-permeable tubing systems, and are seen as a model format that better mimics the in vivo BBB environment, allowing co-culture of NVU cell types, cell-cell contact and replication of BBB microvasculature. Additional benefits include TEER compatibility and the possibility to microscopically visualize the cells within the chip.
- Templated perfusable models. These models involve the gelation of ECM around a template that is designed to mimic functional microvascular tubes. These models replicate shear stress, recapitulate 3D microvessel geometry and allow dynamic reorganization of co-cultured cells as well as real-time permeability measurements, however they are tedious to generate and are not amenable to TEER studies.
That was it for Part 2 of our BBB series. We presented the currently available in vitro methods to study the BBB, but many questions remain unanswered! For instance, what about cell source and quality? Should they always be of human origin and is it better to work with healthy or disease-relevant cells? How can we ensure consistency and minimize donor-to-donor variability when we use donor cells to build a BBB model? And when it comes to commercially available BBB cell models, how do we account for genetic mixtures that exist in pools of cells originating from different donors? Stay tuned for Part 3 where we will address all of these questions!
In the meantime, if you have a question about what you’ve read in our BBB so far, please get in touch with us.
References
- Enabling Novel Treatments for Nervous System Disorders by Improving Methods for Traversing the Blood–Brain Barrier: Proceedings of a Workshop. Washington (DC): National Academies Press (US). 2. Traversing the Blood–Brain Barrier: Challenges and Opportunities. 2018. National Academies of Sciences, Engineering, and Medicine; Health and Medicine Division; Board on Health Sciences Policy; Forum on Neuroscience and Nervous System Disorders. Pubmed Bookshelf ID: NBK507360.
- Williams-Medina A, Deblock M, Janigro D. In vitro Models of the Blood-Brain Barrier: Tools in Translational Medicine. Front Med Technol. 2021. 2:623950. doi:10.3389/fmedt.2020.623950.
- Chin E, Goh E. Blood-brain barrier on a chip. Methods Cell Biol. 2018. 146:159-182. doi:10.1016/bs.mcb.2018.06.003.
- Jamieson JJ, Searson PC, Gerecht S. Engineering the human blood-brain barrier in vitro. J Biol Eng. 2017 Dec 4. 11:37. doi: 10.1186/s13036-017-0076-1.
- Yan Liang, Jeong-Yeol Yoon. In situ sensors for blood-brain barrier (BBB) on a chip. Sensors and Actuators Reports. 2021. 3: 100031. doi.org/10.1016/j.snr.2021.100031.
- Cucullo L, Hossain M, Puvenna V, Marchi N, Janigro D. The role of shear stress in Blood-Brain Barrier endothelial physiology. BMC Neurosci. 2011. 12:40. doi: 10.1186/1471-2202-12-40.
- Stone NL, England TJ, O’Sullivan SE. A Novel Transwell Blood Brain Barrier Model Using Primary Human Cells. Front Cell Neurosci. 2019. 13:230. doi:10.3389/fncel.2019.00230.
Karen O’Hanlon Cohrt is an independent Science Writer with a PhD in biotechnology from Maynooth University, Ireland (2011). After her PhD, Karen relocated to Denmark where she held postdoctoral positions in mycology and later in human cell cycle regulation, before moving to the world of drug discovery. Karen has been a full-time science writer since 2017, and has since then held numerous contract roles in science communication and editing spanning diverse topics including diagnostics, molecular biology, and gene therapy. Her broad research background provides the technical know-how to support scientists in diverse areas, and this in combination with her passion for learning helps her to keep abreast of exciting research developments as they unfold. Karen is currently based in Ireland, and you can follow her on Linkedin here.