Trogocytosis, from trogo meaning ‘to gnaw’ or ‘to nibble’ in Ancient Greek, is an active process during which one cell receives cell surface molecules and membrane fragments from another cell in a unidirectional and cell-contact dependent manner.
Within immune cells, trogocytosis is triggered by receptor–ligand interactions and can occur within minutes of conjugate formation between two live cells. Although the names sound similar, trogocytosis is distinct from phagocytosis, which involves complete engulfment of one cell by another.
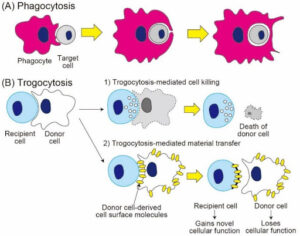
Phagocytosis (A) involves complete engulfment of target cells, while trogocytosis (B) involves partial “nibbling” of donor cells, leading to either trogocytosis-mediated cell death or trogocytosis-mediated material transfer of surface molecules and membrane patches. Image credit: Reference 1, and shared here under Creative Commons Attribution 4.0 International.
An ancient, multifunctional process
The term trogocytosis was coined by Denis Hudrisier and Etienne Joly in 2002 (1). However, the first reports of trogocytosis emerged in the 70s, when a microbiologist in Scotland discovered that the so-called brain-eating amoeba Naegleria fowleri nibbles mouse-embryo cells in a piecemeal fashion rather than swallowing them whole (2). Research carried out since that discovery indicates an ancient evolutionary origin for trogocytosis; it occurs across a broad range of organisms from protozoa to humans and the underlying cellular mechanism is primitive.
Within the immune system, trogocytosis has been observed in T cells, B cells, platelets, macrophages, dendritic cells, natural killer (NK) cells, neutrophils, basophils, innate lymphoid cells, and microglia. However, while most extensively studied in immune cells, it has also been observed in non-immune cells including lymph node stromal cells and mesenchymal stromal cells.
Outside of the immune system, trogocytosis has been observed in neurotransmission, cell-cell communication, stem cell maintenance, development and cellular plasticity, and recent research suggests that it is also used by vaginal neutrophils to eliminate excess sperm from the vaginal lumen without triggering mucosal immunity (3, 4).
Why is trogocytosis important in the immune system?
Trogocytosis is triggered in the immune system by receptor-ligand interactions including T cell receptor (TCR)-MHC interactions between T cells and antigen-presenting cells, Fcγ receptor (FcγR)-interactions with antibody-bound targets, and natural killer cell receptor interactions with target cells.
Trogocytosis can modulate immune function in both stimulatory and regulatory ways that are not fully understood, but can be briefly summarized as:
- T cells acquiring MHC and co-stimulatory molecules from antigen-presenting cells (APCs)
- Natural killer (NK) cells acquiring inhibitory ligands from target cells
- Neutrophils/macrophages killing antibody-opsonized tumor cells (trogocytosis-mediated cell death)
- Non-APCs such as basophils acquiring antigen-presenting ability
- Elimination of microbial pathogens
This can have many different consequences, depending on what material is transferred, including the acquisition of new functions by recipient cells, loss of function(s) by donor cells, and in some cases the death of donor cells in a process known as trogoptosis.
Given its involvement in immune regulation and the fact that it can be directly exploited by certain intracellular bacterial pathogens to spread between cells, a deeper understanding of the mechanisms underlying trogocytosis could lead to new therapeutic avenues for pathogenic diseases, cancer, immune disorders as well as non-immune disorders.
It’s not all positive! Trogocytosis can jeopardize monoclonal antibody therapies
Therapeutic monoclonal antibodies (mAbs) are used to treat various diseases, including cancer and autoimmune disorders. While this therapeutic class is generally considered to be a ground-breaking addition to the medical toolbox, a number of mAbs, in particular those designed to target CD20, have been reported to cause trogocytosis leading to reduced mAb efficacy.
How does this happen? After administration of mAbs, FcγR-expressing cells – such as monocytes, macrophages, neutrophils, and NK cells – can rapidly extract mAb-bound cell surface molecules from target cells via trogocytosis. This results in the target antigen being stripped from the tumor cells, leading to tumor resistance to the therapy. This has been a major limitation of rituximab (anti-CD20) in the treatment of chronic lymphocytic leukemia (CLL).
Several other therapeutic mAbs have been reported to cause trogocytosis, including ofatumumab and obinutuzumab (both anti-CD20) epratuzumab (anti-CD22) , daclizumab (anti-CD25), cetuximab (anti-EGFR), trastuzumab (anti-HER2), and daratumumab (anti-CD38). A deeper understanding of the signaling pathways and mechanisms underlying this will be critical in maximizing the therapeutic benefit of mAbs.
What about trogocytosis in the liver – could that also impede the success of immunotherapies?
CD47 is a major target in immunotherapies against hematologic and solid cancers, but therapeutic programmes to block CD47 have been plagued with failures linked to low efficacy, adverse reactions and patient deaths. A notable example is Gilead’s magrolimab, which was shelved earlier this year following multiple clinical trials in blood and solid cancers, including a failed Phase 3 trial in which magrolimab was linked to patient deaths.
The rationale for targeting CD47 is to block a so-called ‘don’t eat me signal’ that cancer cells express in order to avoid being phagocytosed. This signal inhibits neutrophil trogoptosis via interaction with signal regulatory protein a (SIRPa). So, targeting CD47, e.g., with an anti-CD47 mAb should block that ‘don’t eat me’ signal and result in augmented cancer cell trogoptosis.
One other possibility that has not been studied in detail is that anti-CD47 antibodies might be trogocytosed into liver cells; this would further reduce their therapeutic impact and contribute to a reduced therapeutic window where efficacy is too low to justify side effects. In support of this idea, trogocytosis has been observed in several liver cell types, including liver-resident immune cells, Kupffer cells, and liver sinusoidal endothelial cells (LSECs). Unfortunately, most pre-clinical toxicology studies only look at the HepG2 cell line (isolated from a person with hepatocellular carcinoma in 1975), which like other immortal cell lines can accumulate mutations over time in culture, exhibit unique gene expression signatures and not display the phenotypes, attributes or functions of in vivo hepatocytes. Since LSECs and Kupffer cells are not usually included in pre-toxicity studies at all, trogocytosis of therapeutic antibodies into these cell types remains a possibly overlooked cause of liver toxicity.
We have previously discussed the pros and cons of working with immortalized cell lines, and highlighted human induced pluripotent stem cells (hiPSC) as an alternative to such cell sources in drug discovery and development. Advances within hiPSC technologies in recent years have made it possible to generate reproducible and scalable human-relevant cell resources, including various liver cell types that can be cultured together to generate human liver-like 3D organoids, which you can read much more in a recent Tempo article here.
Stay tuned for more articles on liver cell types, how they vary between humans and animals, and how these differences can impact drug development and success!
References:
- Hudrisier D. & Joly E. Plasma membrane nibbling: all lymphocytes do it, but why? ELSO Gaz. 9, 1–5 (2002).
- Brown T. Observations by immunofluorescence microscopy and electron microscopy on the cytopathogenicity of Naegleria fowleri in mouse embryo-cell cultures. J Med Microbiol. 1979 Aug;12(3):363-71.
- Olivera-Valle I., Latorre MC., Calvo M, et al. Vaginal neutrophils eliminate sperm by trogocytosis. Hum Reprod. 2020 Nov 1;35(11):2567-2578.
- Uribe-Querol E. & Rosales C. The Multiple Roles of Trogocytosis in Immunity, the Nervous System, and Development. BioMed Research International, 2021, 1601565, 16 pages, 2021.
Karen O’Hanlon Cohrt is an independent Science Writer with a PhD in biotechnology from Maynooth University, Ireland (2011). After her PhD, Karen relocated to Denmark where she held postdoctoral positions in mycology and later in human cell cycle regulation, before moving to the world of drug discovery. Karen has been a full-time science writer since 2017, and has since then held numerous contract roles in science communication and editing spanning diverse topics including diagnostics, molecular biology, and gene therapy. Her broad research background provides the technical know-how to support scientists in diverse areas, and this in combination with her passion for learning helps her to keep abreast of exciting research developments as they unfold. Karen is currently based in Ireland, and you can follow her on Linkedin here.